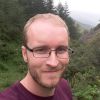
Dr Craig Magee
- Position: Independent Research Fellow
- Areas of expertise: magma emplacement; sills; dykes; normal faults; seismic reflection; fieldwork; anisotropy of magnetic susceptibility; structural geology; volcano deformation
- Email: C.Magee@leeds.ac.uk
- Phone: +44(0)113 343 6500
- Location: 7.20 Priestley Building
- Website: Twitter | Googlescholar | ORCID
Profile
As a structural igneous geologist I specialise in applying different techniques to study the structure and emplacement of magma plumbing systems, and their effect on ground deformation. I was recently awarded a NERC Pushing the Frontiers grant as PI to investigate how magma emplacement translates into ground movement, specifically focusing on incorporating realistic rock mechanic behaviours into volcano deformation models. I joined the University of Leeds in October 2018 after being awarded a NERC Independent Research Fellowship (IRF) to understand "How do faults grow above dykes?", which involved using seismic reflection data and LiDAR imagery to examine dykes and dyke-induced normal faults in 3D. This project built on my previous research at Imperial College London where, as a Post-doctorate Research Associate (2011-2015) and an Imperial College Research Fellow (2015-2018), I developed expertise in using seismic reflection data to image the structure of sills and sill-complexes, relating surrounding host rock deformation to magma emplacement mechanics. Fieldwork is a fundamental aspect of my research and formed the basis of my PhD, completed at the University of Birmingham (2008-2011), on "Emplacement of sub-volcanic cone sheet intrusions". Beyond studying the structure and emplacement of magma plumbing systems, I have interests in: (i) volcano growth and deformation; (ii) geometry and growth of normal faults; (iii) internal deformation within salt bodies; (iv) magnetic characterisation of salt; (v) magma-salt interactions; (vi) basin development; and (vii) the role of magma in plate tectonics through time.
Awards and funding
- NERC Pushing the Frontiers grant (2023)
- Fellow of the Higher Education Authority (2022)
- Fowler Award (Geophysics) - Royal Astronomical Society (2020)
- NERC Independent Research Fellowship (2018)
- Murchison Fund - Geological Society of London (2016)
- Halstead Award - Geologists Association (2016)
- Imperial College Research Fellowship (2015)
- Shell Prize - Tectonic Studies Group conference (2010)
- Mike Coward Prize - Tectonic Studies Group conference (2010)
PhD Co-supervisor
- Jonas Kopping (2018-2022) - From planes to pipes: The emplacement of elongate intrusions in shallow magma plumbing systems
- Vincent Twomey (2017-2022) - Shallow-level granite emplacement in Iceland - University of St Andrews
- Jack Richardson (2016-2019) - Magnetic characterisation of salt deformation - University of Birmingham
- Murray Hoggett (2014-2019) - Sill emplacement mechanics - University of Birmingham
- Matthew Reeve (2014-2017) - Tectono-stratigraphic evolution of the NW Australian Shelf - Imperial College London
Research interests
My research interests primarily focus on understanding how magma is emplaced and accommodated within the crust. Here, I list and describe several ongoing areas of research:
MAGMA: Magma Accommodation and Ground Movement Analysis (NERC Pushing the Frontiers)
MAGMA will transform how we capture the complex geology beneath volcanoes within the numerical models of volcano ground movement that are used in eruption threat assessment; this will help drive significant improvements in forecasting eruptions, helping keep people safe and secure.
Over 800 million people live near volcanoes. To keep people safe and secure, we need to reliably forecast when volcanic eruptions may occur and what their potential size, style, and hazards will be. However, because the geology and plumbing system dynamics beneath each volcano is unique, all volcanoes behaves differently, making it difficult to reliably forecast eruptions. As magma intrudes through the crust and accumulates, it often pushes up the overlying rock and Earth's surface. Ground movements at active volcanoes thus often herald eruption. We therefore monitor the surface elevation of volcanoes, using satellites and ground-based tools, to look for tell-tale ground movements related to magma build-up. Using sophisticated numerical models, we can estimate the amount and location of magma required to drive measured ground movement. These estimates of magma bodies provide crucial inputs for eruption forecasts as they constrain how close magma may be to the surface, its volume and pressure, and how fast it is moving.
A key flaw of many ground movement models is that they assume the rocks through which magma moves are simple and have no internal structure or compositional variation. Yet we know rocks vary physically and chemically at all scales, and how they deform changes in space and time. Critically, ground movement models that include more realistic geology (e.g. layering) show that, incorporating even small degrees of complexity can change estimated magma body properties by orders of magnitude. Such changes in magma body estimates may be the difference between forecasting an eruption or not.
Reliably using ground movement to forecast volcano eruption onset, size, style, and hazards requires models that realistically capture host rock complexity. For example, uplift above injecting magma requires the overlying rock to bend. Yet we actually know very little about how resistant rocks are to bending. We also do not know how local extension and compression within the bending rock volume changes its material properties and thus affects its response to further deformation. Critically, these controls on rock bending dictate how much and where ground movement occurs above injecting magma. To solve these problems in MAGMA, we will:
1) Conduct mechanical experiments where we load and bend different rocks to measure their resistance to bending; lab results will be 'upscaled' using tried and tested geotechnical methods so they are representative of entire rock masses.
2) Examine the geometry of and deformation within ancient areas of uplift above magma bodies exposed at the surface or imaged in 3D seismic reflection data, which provides ultrasound-like images of the subsurface.
3) Collect samples from field areas and use mechanical experiments to pull and compress them in the lab to test how bending locally changed their resistance to deformation.
MAGMA will use this information to build novel Finite Element numerical model that can capture multiple aspects of host rock complexity. These models will adopt a perturbation theory approach, which has been successfully tried and tested in complex ground movement models of earthquakes. With our developed method, we will create synthetic but realistic
[taken from NERC proposal summary]
Collaborators: Dr James Hickey, Dr Susanna Ebmeier, Prof. John Forth, Dr Janine Kavanagh, Dr William McCarthy, Dr John Browning, Prof. John Howell
How do faults grow above dykes? (NERC IRF project – completed)
When dykes are injected, either vertically or laterally, they fracture and push apart the surrounding rock, producing small earthquakes. Continued dyke injection causes fractures to develop into faults, where rock on one side of the crack starts to slip passed the other. Fault slip can pull down and extend or push up rock directly above the dyke, sometimes deforming Earth's surface. Monitoring earthquakes and ground deformation generated by dyke-induced faults can therefore tell us where dykes are injecting, providing us warning of possible eruptions. Studies of injecting dykes and dyke-induced faulting in Ethiopia show they can also aid continent fragmentation, although these structures have yet to be found along the margins of continents where break-up once occurred. In addition, satellite images of planets (e.g. Mars) indicate dyke-induced faults deform their surface. It is thus clear that dyke injection and dyke-induced faulting plays and has played a major role in shaping the volcanic and tectonic history and surface morphology of Earth and other planets.
To understand how dykes and dyke-induced faults control different volcanic, tectonic, and planetary processes, we first need to identify how faults grow above dykes in three-dimensions. However, seismicity and ground deformation related to active dyke injection, which cannot directly be observed, are rarely captured using geophysical techniques and only a small part of a dyke-induced fault can be studied at the surface. Conversely, where ancient dykes are exposed at Earth's surface, erosion of the overlying rocks has often removed dyke-induced faults and the earthquakes that accompanied dyke injection have long-since ceased. To circumvent these problems, many computer and sandbox models have been developed to try and replicate fault growth above dykes. These models have produced numerous hypotheses for dyke-induced fault growth, but without examination of the 3D structure of natural dykes and dyke-induced faults, they cannot be tested. Therefore, despite over 40 years of research, we still do not understand the true 3D structure or evolution of dykes and dyke-induced faults.
I have identified a series of ancient dykes and dyke-induced normal in seismic reflection data, which provide 3D X-ray like images of Earth's subsurface, from the margins of a continent (NW Australia). These data present a unique and exciting opportunity to study the 3D structure of dykes and dyke-induced faults. By measuring offset of sedimentary rocks across faults, which record how slip accumulated, I test previous model predictions of dyke-induced fault growth. Because the processes driving dyke injection and faulting offshore of NW Australia have long since ceased, I also study active dyke-induced faults breaking the surface in Ethiopia. I specifically use high resolution, aerial Light Detection and Ranging (LiDAR) images collected in 2009 and 2012 to identify how faults grew and interacted during a single dyke injection event in 2010. Results from these analyses will be used to design of new analogue models that will replicate dyke injection and dyke-induced faulting in 3D, under different tectonic settings (e.g. extension), and using more realistic rock/magma characteristics. This cross-disciplinary research will reveal how faults grow above dykes, raising important implications for our understanding of: (i) how we can use dyke-induced fault activity to assess potential eruptions; (ii) the role dykes and dyke-induced faults play in the break-up of continents; (iii) whether dykes and dyke-induced faults influence the evolution of continental margins; and (iv) dyke and fault structure beneath the surface of other planets (e.g. Mars).
[taken from NERC proposal abstract]
Collaborators: Prof. Tim Wright; Prof. Chris Jackson
How are sill-complexes constructed?
Textbooks suggest volcanoes are underlain by dykes, where magma predominantly moves vertically through the crust. However, recent studies champion a new idea where the lateral extent of magma plumbing systems is greater than their vertical extent, with magma primarily transported in a network of sub-horizontal sills (i.e. a sill-complex) as opposed to dykes. Seismic reflection data can image sill-complexes in 3D, revealing they comprise interconnected networks of sills and inclined sheets, typically intruded within sedimentary basins, but also crystalline basement. The Karoo Sill-complex (South Africa), spanning an area the size of Spain, and the Ferrar Sill-complex (Antarctica), which extended horizontally for >4000 km, provide excellent examples of such laterally extensive magma plumbing systems. Field observations and seismic reflection data have allowed the broad structure of sill-complexes to be constrained and shown how individual intrusions are emplaced. However, we do not know how entire sill-complexes are built and transport magma over large areas without freezing. It has been suggested that hot but solidified sections of sill-complexes allow later magma injections, focused into channels or along sill boundaries, to flow further and gradually extend the sill-complex. A key interest of mine is to test these ideas by investigating how magma moves through sill-complexes, using a range of techniques, with a view to understanding how magma flow pathways influence the distribution, construction, and eruption of volcanoes.
Collaborators: Prof. Chris Jackson; Dr William McCarthy
Forced folding above shallow-level intrusions
Space for shallow-level sills and laccoliths is commonly generated by bending and uplift of overlying rock and sediment. This so-called ‘roof uplift’ produces forced folds, the shape and amplitude of which reflect the geometry of underlying intrusions. The surface expression of forced folds can therefore be inverted to constrain intruding magma body properties, whilst ancient forced folds provide a record of sill and laccolith emplacement. Deciphering how shallow-level intrusion translates into roof uplift is critical to enhancing our understanding and forecasting of magma emplacement. Whilst my research centres around quantifying the geometry of intrusions and overlying forced folds in 3D using seismic reflection data, I am also working on understanding these systems through fieldwork and analogue modelling.
Collaborators: Prof. Chris Jackson; Dr William McCarthy; Prof. Giacomo Corti; Dr Domenico Montanari
Other research interests
- The role of sills in continental break-up
- Continent-to-ocean transition in the Danakil Depression, Ethiopia
- Emplacement and accretion of sheet intrusions in Iceland
- Emplacement of the Scourie Dykes and implications for the role of dykes and dykes swarms in early Earth tectonics
- Magma-salt interactions
- Characterising deformation in salt bodies using rock magnetic techniques
- Tectono-stratigraphic evolution of NW Australia
- Magmatism and volcanism offshore New Zealand
Qualifications
- PhD, Geology, University of Birmingham
- MSci, Geology, University of Birmingham
Professional memberships
- Fellow, Geological Society London
- Member, Geologists Association
- Member, Volcanic and Magmatic Studies Group
- Member, Tectonic Studies Group
Research groups and institutes
- Volcanology
- Basin Structure
- Geodynamics and Tectonics
- Institute of Geophysics and Tectonics
- Institute of Applied Geoscience